Supervised by: Aparajita Sriram, MSc, MPhil. Aparajita is currently completing an MPhil degree in genomic medicine at the University of Cambridge, and is looking forward to starting a research position at the University of Exeter to look at the genetic basis of rare types of diabetes. She is particularly interested in circadian rhythms and psychiatry.
ABSTRACT
As cancer becomes more prevalent in society due to lifestyle choices and environmental factors, the rise in tumour relapses has led to the discovery of tumour-initiating cancer stem cells (CSC), which show the properties of self-renewal, heterogeneity and differentiation. Yet, as the medical field designs CSC-terminating drugs to eradicate metastasis, in vitro experiments have shown CSCs’ ability to drive cellular anti-apoptosis features and tumorigenic transcription, as well as efflux anti-cancer drugs. Thus, putting pressure on modern cancer treatment strategies like chemotherapy, radiotherapy, immunotherapy and CAR T-cell therapy. This has led to the evolution of new targeted therapies, to see how effective therapies are to inhibit functions that regulate CSC’s fate under the interference of multidrug resistance, level of heterogeneity, and the constraints regarding finance and time. In this article, we discuss the significant target mechanisms – signalling pathways like Hedgehog, Wnt, Notch, protein complex YAP1, and the ABC transporters, and the level of efficiency they have in achieving the goal of CSC apoptosis. With the medical field seeing publication of successful CSC-targeted drugs, like Trastuzumab, Vismodegib and Metformin, it highlights the role CSCs play in strengthening cancer and the importance of targeting them. Despite their favourable results, improvements are due to be made, including further understanding of CSCs and biomarkers, addressing ethical concerns of clinical trials and overcoming the problems of tumour evolution and heterogeneity.
INTRODUCTION
Cancer
Cancer is a large group of diseases, characterised by the uncontrolled growth and division of cells within the human body (Allott and Mindorff, 2014). For a healthy individual, cells have the ability to grow, divide and undergo apoptosis in a controlled manner, in order to maintain bodily functions and repair tissues. However in the case of cancer, this orderly process is disrupted, producing a population of abnormal cells; as a result, a mass is formed known as a tumour. These cancerous cells have the ability to invade nearby tissues, and in some cases, spread to other parts of the body, through the process known as metastasis (Allott and Mindorff, 2014). The development of cancer is most often driven by genetic mutations, leading to alterations in the normal regulatory mechanisms, which usually control the processes of cell growth, division and death (Sever and Brugge, 2015).
Table 1: The variety of factors, environmental and lifestyle which can cause the production of mutations
Environmental factors:
|
Lifestyle choices:
|
Normal Stem Cells and Cancer Stem Cells
The evolution of cancer treatment is marked by significant advancements. One example includes cancer stem cells, which play a pivotal role in the development of effective cancer treatment plans. These cancer stem cells (CSCs) are a subset of cells within a tumour that have gained the ability to sustain tumour growth (Yu et al., 2012).
In general, stem cells are a unique cell type found within multicellular organisms and are characterised by the ability to develop into various specialised cell types. Through self renewal and potency, stem cells can divide and produce identical copies of themselves, ensuring that there is a continuous population of stem cells within the organism. Stem cells are often classified based on their potential to differentiate into different cell types:
- Totipotent: only the earliest stages of human embryos are totipotent, such as extra-embryonic and embryonic tissues, and can be differentiated into any type of cell (Allott and Mindorff, 2014).
- Pluripotent: these stem cells can differentiate into a variety of stem cells and can form any cell type (Allott and Mindorff, 2014).
- Multipotent: these stem cells are more limited in their differentiation potential, and can differentiate into a number of closely related cell types (adult and somatic stem cells) (Allott and Mindorff, 2014).
- Unipotent: muscle stem cells are an example, and they cannot differentiate, however they are capable of self renewal (Allott and Mindorff, 2014).
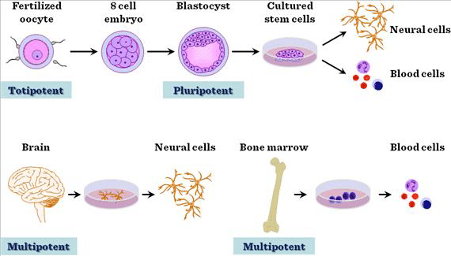
Figure 1. An image to show the stages of potency, and the levels to which stem cells can differentiate and specialise (Domokos Bartis, 2011).
Table 2: Similarities and differences between cancer stem cells and normal stem cells
Similarities between cancer stem cells & normal stem cells (Rahman et al., 2016) |
Differences between cancer stem cells & normal stem cells (Rahman et al., 2016) |
|
|
There is a realm of factors that makes CSC’s so difficult to treat. At the forefront of these problems is a phenomenon called epithelial mesenchymal transformation (EMT), a process by which epithelial cells that usually communicate with one another develop into mesenchymal cells which invade tissues. This pathway involves an increase in resistance to apoptosis, thus allowing the cancer stem cell to avoid death, and an increase in extracellular matrix components (Dave et al., 2012). Recently, studies have shown that EMT is responsible not only for tumour metastasis, but also tumour recurrence and drug resistance. An increase in EMT markers has been linked to an increase in aggressive metastasis and intrinsic resistance to standard therapies, therefore posing a barrier to current treatment strategies (Kong et al., 2011).
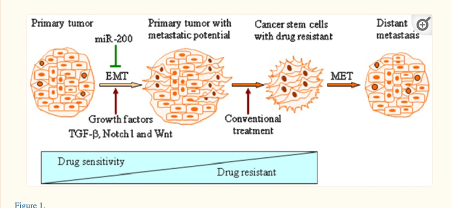
Figure 2: Induction of epithelial-to-mesenchymal transition (EMT)-phenotypic cells produces cancer stem-like cells with drug-resistant characteristics
The figure above demonstrates the contribution EMT makes to producing cancer stem cells with multi-drug resistance. Another reason why CSC’s are so hard to treat is due to the nature of the tumour. Quiescence is a major factor in challenges with cancer therapy, as it is a state in which the cancer stem cells are not dividing rapidly. Thus, modern therapies designed to attack rapidly-dividing cells have little effect (Chen et al., 2016b).
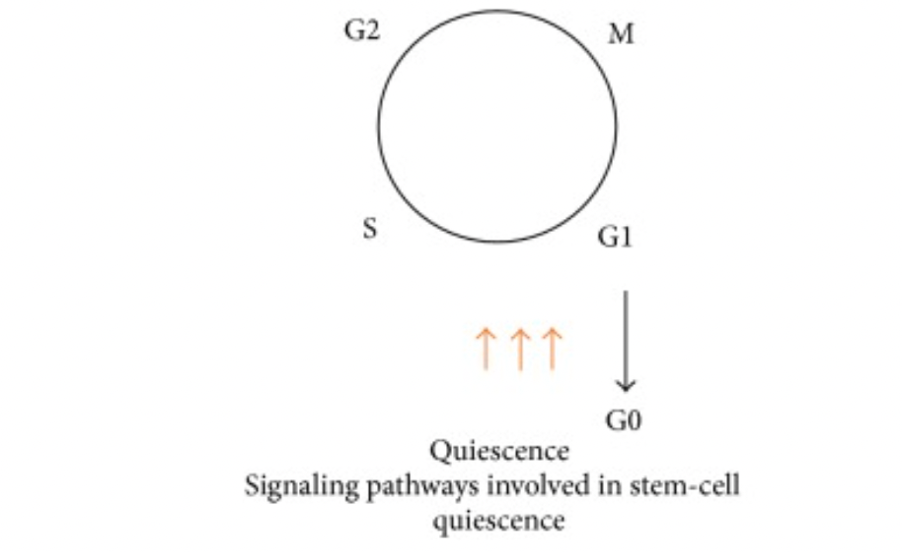
Figure 3: The properties of cancer stem cells, such as the signalling pathways on their surface, contributes to their quiescence and thus unresponsiveness to treatments (Chen et al., 2016b).
Going forward, neutralisation of this quiescent phenotype is a promising treatment for anticancer protocols.
Lastly, another feature of CSC’s that provides a barrier to effective treatment is the overexpression of anti-apoptotic molecules. Apoptosis is essentially cell death, a tightly regulated, programmed suicide, which is harnessed usually when mutations in a cell have accumulated leading to its ill function. In brain-tumour derived CSC’s, due to higher levels of survivin, belonging to the IAP (inhibitors of apoptosis), the tumour was resistant to treatments targeting its apoptotic pathways to induce death – so the tumour survived and the treatments were ineffective (Chen et al., 2016).
REVIEW OF LITERATURE
Modern cancer treatment strategies
Chemotherapy
The treatment utilises drugs to cease the proliferation of cancer by killing fast-growing cancer cells. These chemotherapeutic drugs include antimetabolites (prevent renewal of cancer cell genetic material), alkylating agents (damages cancer cell DNA to prevent proliferation), and topoisomerase inhibitors (stop cancerous DNA from duplicating). They can be injected intravenously (directly into a vein), taken orally or by intra-arterial injection (injected directly into the artery that holds metastatic material). Yet, aside from their main purpose, they terminate healthy cells, causing side effects like hair loss, nausea or fatigue. Hence, older patients tend to pull back from chemotherapy as it increases the chance of organ failure to cause death (NIH, 2022).
Radiotherapy
The ongoing treatment of radiotherapy relies on high doses of radiation to exterminate the spread of cancer by damaging its DNA. External beam (applies local treatment using a machine) and internal beam (putting a source of radiation into patients’ bodies) are the two major radiotherapy types, which are painless processes to ease cancer proliferation. Yet, the radiation is widespread and affects normal working cells. Hence, there is a lifetime dose limit to prevent death from excessive intake of radiation (NIH, 2022).
Immunotherapy
Immunotherapies therapy utilises the patient’s immune system to fight cancer, with some showing promising results even against CSCs. For example, the engineered protein, monoclonal antibodies (MAB), are designed specifically to bind with antigens found on the surface of CSCs, recognising to eliminate them while leaving the normal cells intact. There are many ways by which MAB target CSC:
- By identifying the unique CSC biomarkers: MAB binds to the unique surface markers or molecules on the CSCs to locate and eliminate them.
- Through self-renewal pathways: As CSCs rely on their self-renewal trait to maintain their population in a cell, MABs can be engineered to disrupt these pathways and inhibit this ability by isolating the unique molecules found in the self-renewal pathways.
- Through antibody drug conjugates: Antibody drug conjugates (ADC) are MABs embedded with cytotoxic drugs. These ADCs then distribute a lethal load of cytotoxic drugs directly to CSC while not harming healthy cells or tissue. An example of an effective ADC is Trastuzumab, which is a drug that treats the early stages of breast cancer. (NIH, 2022).
CAR T-cell therapy and biomarker testing
Diagnosis and drug prescription for cancer patients have been categorised by understanding the biomarkers of cancer cells, which help to predict the progression of tumours for modification of treatment strategies. This personalised medicine collects patients’ T-cells (immune cells – a type of white blood cell) and is modified with CARs to be infused into the bloodstream, where CAR T–cells attach to terminate cancer cells. The inhibition of cancer relapse has seen a success rate of 76%, which shows the modified T-cells’ persistence to cancerous potential (NIH, 2022).
Examples of successful anti-cancer drugs
Despite the heterogeneity and high resistance of the CSC, drugs have been designed to reduce the challenge by initiating targeted therapies that inhibit key growth mechanisms in the CSC to function.
Trastuzumab
This drug, also known by the trading nameHerceptin, treats non-spread early breast cancer or spread breast/stomach/gastric junction cancer. Discovery was due to the recognition of the biomarker, HER2 (human epidermal growth factor 2), which was widely expressed in a type of CSC. The ligands from stromal cells bind to the HER2 receptors to signal for renewal. Hence, trastuzumab uses monoclonal antibodies to clog the receptors and stop cell transduction, transcription (first step to gene expression) and proliferation. Experimental patients have achieved a disease control rate of 97.3% and patients who have intaken 1 year of trastuzumab had a 5-year disease-free survival rate of 87.9% (Cancer Research UK., 2021).
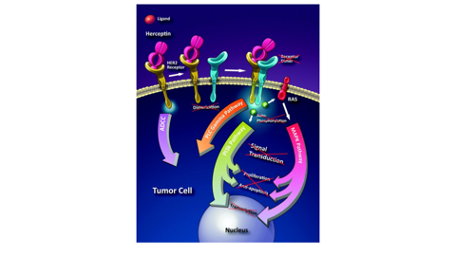
Figure 2.1: How trastuzumab works to terminate CSC (Gemmete & Mukherji, et al. 2011).
GDC-0449 (Vismodegib) and LDE225 (Sonidegib)
Both Vismodegib and Sonidegib are used to treat patients with metastasized basal-cell carcinoma by preventing further tumour spread and aiding shrinkage of tumours. They function as Hedgehog pathway inhibitors that block the proliferation signals by binding to the Smo protein (mediates signal transduction in the Hh pathway) on the Hh ligand cell surface receptors.
- The estimated disease control rate of Vismodegib from the STEVIE trial, a multicentre study to reassure the safety of Vismodegib, is 92.9% , which is a highly promising result (Broderick, et al. 2020). The side effects include cramps, pain and muscle stiffness, which will not require intense medical attention.
- The estimated 2-year survival rate of Sonidegib is 93% with common side effects of diarrhoea, vomiting and loss of appetite, which might be more severe than Vismodegib. Yet, they are not life-threatening effects, allowing the drug developers to anticipate the publication of the drug after further improvement.
Metformin
This is an anti-diabetic agent that was proven to eliminate CSCs with the aid of the chemotherapeutic agent, doxorubicin(Daugan, 2016). From in vitro and in vivo experiments with weaker CSC, it is found that the drug blocks off the mTOR pathway, which is important in regulating the CSC cycle and directly acts to inhibit tumour angiogenesis or metabolism. Its side effects include nausea, insomnia, and vomiting, which will require medical attention if worsened (Marie Daugan et al., 2016). Yet, no impact has been shown on advanced pancreatic cancer during randomised studies in 2015; hence, further refurnishing has to be done to ensure a higher success rate.
The theory and mechanism of target therapy
Target Therapy
Chemotherapy and radiotherapy are unable to directly target CSCs because of their evolutionary capabilities. In order for therapies to be effective, stronger therapies must be invented targeting signalling pathways and biomarkers, thus eliminating or slowing down the reconstructive properties of CSCs.
CSCs can divide and replicate themselves. By differentiating, CSCs leave more and more biomarkers behind. CSCs have unique markers that they share with other stem cells and of their own. These unique biomarkers on CSCs can be used to identify and isolate CSCs, as well as monitor the activity of the CSCs, which can then be used to predict tumour growth. This may potentially lead to tumour resistance.
Target mechanisms include:
Signalling pathways: The Wnt, Hedgehog and Notch signalling pathways are critical developmental signalling pathways that regulate cell proliferation and induce the effect of anti-apoptosis in CSCs.
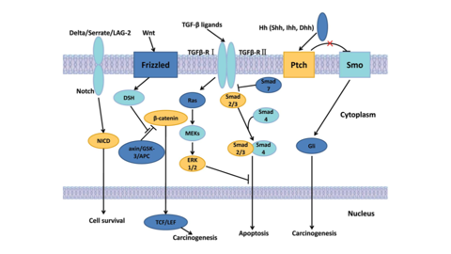
Figure 2.2 the compilation of the most important signalling pathways (Song et al., 2013).
Wnt signalling pathway
The Wnt signalling cascade maintains tissue regeneration to regulate the stemness of CSC. Yet, misexpression of the Wnt ligands, like the nuclear beta-catenin (regulation of gene transcription) expression, makes it liable to the creation of a cancerous cell population (Zhou et al., 2021). Signalling is induced when the Wnt proteins bind to the frizzled (Fz) family receptors on the cell membrane, with aid from co-receptors like LPR-⅚ (lipoprotein receptor-related protein → used in the receptor-mediated process of transporting substances) or RTK (receptor tyrosine kinase → initiates signals for foreign substance bonding). After activation of the receptor, signals are sent to the phosphoprotein Dishevelled (Dsh → transduces signals into the three main Wnt signalling branches: canonical, non-canonical and Ca2+), where Dsh proteins are present to communicate with the different domains (Kim & Kahn, 2014). Hence, drugs should be designed to occlude the Dsh site or to put an early halt to the interaction of the Wnt proteins and the Fz receptors.
Hedgehog signalling pathway
The Hedgehog pathway plays an important part in the developmental biology of organ tissues and transmits cell differentiation signals to embryonic cells. Yet, the false activation of Hh signals can lead to the resistance of CSC. The Hedgehog pathway regulates the gene transcription associated with angiogenesis and metastasis of CSC, preserved by the protein complex, mTORC2, retaliating the growth factors for stable production of Gli2 protein which is vital to metastasis (Davis, 2020).
The pathway’s signal transduction occurs when the Hh ligand produces the sonic hedgehog protein to bind with the Ptch1/2 receptors (tumour suppressor gene). Ptch inhibits the production of Smo (protein to regulate cell survival) to activate the Gli2 proteins, which move into cell nuclei to transcript proliferation (refer to figure 1.1) (Cochrane et al., 2015). This process sorts cell-enhancing signals to different pathway activity levels to induce stemness. Hence, it is crucial that future cancer therapies implement Hh pathway inhibitors to abolish CSC’s self-renewal characteristic. The drugs should be designed to either prevent the mutation of the Hh ligand or to block off receptors from receiving proliferation signals by binding with Hh ligand.
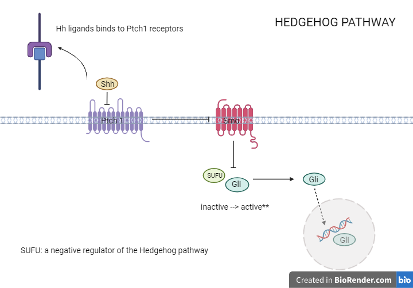
Figure 2.3: How signal transduction occurs in the Hedgehog pathway
Notch signalling pathway
This pathway regulates the communication between cells, contributing to embryogenesis normally and signals of differentiation during oncogenesis. It possesses the 4 receptors (NOTCH1/2/3/4) and ligands (JAG1/2 and DLL1/3/4), where these transmembrane components bind the NICD (notch intracellular domain) and notch extracellular domain. Signalling is induced when the activated DLL (delta-like ligand → induce tumour angiogenesis) binds to the extracellular domain of the notch receptors, activation processed by the MIB protein (Mindbomb1). S2 cleavage occurs as the protease Adam shifts the component of the Notch receptor off the receiving cell, then S3 cleavage happens when the secretase gamma cleaves NICD off the notch receptor. The NICD is now free in the cytosol and binds to complexes in charge of transcription factors like CSL and P300, which are transferred into the nucleus to transcript notch targeted gene (JJ Medicine, 2017).
This activation is shown to induce self-renewal and anti-apoptosis features in CSC, proven by the exemplar of the T-ALL model (T lineage acute lymphoblastic leukemia). The NOTCH1 target, CD44, was found present in pre-leukemic cells, activating mutations of the NOTCH1 gene to play a key role in clonal dominance. As cancerous cells rely on communication between the stromal microenvironment and leukaemia cells, this increases the number of notch receptors and the ligands (i.e. DLL1), causing the maintenance of human T-ALL cells (Meisel et al., 2020).
Another possible hypothesis for the notch signalling pathway to be increasing cancer potential is due to the mechanism of the FBW7 (F box and WD repeat domain-containing 7). This is a secreted E3 ubiquitin ligase, which means its functions are to aid the NCID to shut down its transcription process and produce degradation of the domain after safe levels of gene copying. Yet, if the FBW7 is not able to function or is weakened to not be present, the NCID might carry out excessive transcription, causing mutated differentiation, which could be a reason why proliferation can be induced by the Notch signalling pathway.
YAP1 protein
The effector of the Hippo signalling pathway, YAP1 (yes associated protein 1), is a transcriptional regulator that regulates cellular survival and overpowers the apoptosis genes. Recent studies on cancer drug resistance, like breast, bladder and lung cancer, have shown that it contributes to the maintenance of a resistant tumour microenvironment. It is able to reprogramme non-cancer cells to have the stemness of a CSC, regulating this pluripotency by interacting with other transcription factors like OCT4, SOX2 and various oncogenic pathways like Hippo, and mTOR. It works alongside the activated Wnt signalling pathway to induce release into other cell nuclei, where the beta-catenin and YAP1 genes can see an increased rate of expression. Eliminating this protein by altering its environment or by targeting the pathway that activates its functions will reduce the rate of metastasis (Shibata & Hoque, 2019).
Verteporfin is a drug to treat blood vessel disorders but acts also as an efficient photodynamic therapy that suppresses YAP1, reducing the tumorigenicity of cells. As the over-expression of YAP1 is induced by SOX2, which is activated by COX2 signalling, this drug acts alongside the COX2 inhibitor to lower the cancerous potential.
ABC transporters (ATP binding cassette)
ABC transporters are proteins that transport substrates across the cell membrane, utilising energy derived from ATP hydrolysis. The overexpression of ABC transporters mediates the efflux of cytotoxic chemotherapeutic drugs out of the cell, strengthening the multi-drug resistance of CSC. Examples include ABCB1, ABCG1 and ABCG2 transporters. The inhibition of the Gli1 protein, which maintains the ABCB1 and the PI3K signalling, regulates ABCG2. Aerobic glycolysis/Warburg effect (occurs in the presence of oxygen) is efficient in producing ATP, which activates the oncogenes and provides a metabolic environment. Poorly perfused areas limit the nutrient supplies, yet CSCs will still generate mitochondrial ATP to survive (Begicevic & Falasca, 2017). Hence, targeted drugs can be designed to limit the level of ATP or inflict hypoxia, which avoids the transport system to reinforce multidrug resistance. Causing the cell membrane to be selectively permeable can also stop the efflux of chemotherapy drugs, but this could influence other molecules to affect other processes of the human body.
Limitations to therapeutic treatments against CSC:
Multidrug Resistance
In CSCs, multidrug resistance (MDR) is one of the leading causes of chemotherapy and radiotherapy failure. Tumour dormancy is a state of cancer that is medically undetectable, which contributes to multidrug resistance as well as many other problems including minimum residual disease, tumour outgrowth, cancer relapse, and metastasis. This causes CSCs to be able to mediate resistance to therapy, which means cells are in the G0-phase, but can still divide during a mitotic stimulation. Yet, MDR has many mechanisms to which it operates (Bukowski, 2020).
One mechanism (cause) of multidrug resistance is elevated metabolism of xenobiotics. Xenobiotics are chemical substances that are found within foreign substances, like the anti-cancer drug. Carrier molecules and enzymes then metabolise the drug to detoxify the chemotherapeutics. These enzymes have an increased expression in cancerous tumours and enhanced detoxification on chemotherapeutics in CSCs (Bukowski, 2020).
Other important mechanisms of MDR are the increased DNA repair capacity and microenvironmental growth factors. Chemical therapeutics attack the DNA within CSCs. The CSCs ability to repair damaged DNA from the chemotherapeutics is accomplished by two mechanisms: homologous recombination repair mechanisms (RRM) and nucleotide excision repair (NER) systems. Growth factors are enabled by hypoxia in the tumour. A couple of growth factors are angiopoietin (Ang)− 2, which is one of the main signalling pathways involved in creating new blood vessels, and platelet-derived growth factor (PDGF), which is a strong activator cells of mesenchymal origin and stimulates chemotaxis and proliferation. Another few growth factors include vascular endothelial growth factor (VEGF), which is a powerful angiogenic factor and is an essential growth factor for vascular endothelial cells, transforming growth factor (TGF)-α, which is a single-chain polypeptide, and hepatocyte growth factor (HGF), which is a strong mitogen for hepatocytes and many other types of cells like endothelial and epithelial cells, melanocytes, and keratinocyte (Bukowski, 2020).
The final mechanism of MDR is genetic factors, such as gene mutations, amplifications, and epigenetic alterations. Gene mutations are considered one of the main causes of the failure of chemotherapy treatment due to the loss or reassortment of chromosomes during mitosis, which are responsible for losing drug-sensitivity genes and changes in biochemical pathways (Bukowski, 2020).
Mutations of the TP53 gene are often seen in tumour cells. Research has shown the TP53 gene protects an organism against tumour progression and regulates the cell cycle and inducing apoptosis. When the TP53 gene is mutated, it loses its tumour suppressive activities and reverses the protective role, initiating chemoresistance, invasion, and metastasis. Anticancer drugs cause DNA damage and cell death by TP53 activity, so losing this activity in cancer cells allows continued proliferation, no matter the type or extent of damage done to the DNA. This causes a high resistance of CSCs to anticancer drugs (Bukowski, 2020).
Heterogeneity of the tumour
The heterogeneity of a tumour is the concept that there are various cell types in one malignant tumour bulk, differing both phenotypically and molecularly. The molecular structure and the microenvironment of the tumour are factors that create variations in CSCs, making it challenging to induce death of CSC by targeting a specific cell as they each respond differently to treatment. When a biopsy is taken from the tumour, the sample may not accurately represent the tumour as a whole. The cell type pulled from the sample may make up only 10% of the tumour, while other cell types could make up around 80%. Medication is prescribed to only target the cell type recognised from the sample, but a different drug would be much more efficient at generating death to the major cell type, which then leads to apoptosis of the CSC. Every tumour comprises of different cell types and hence treatment differs. More biologically and technologically advanced tools are needed to personalise the treatment given to each person (Proietto et al., 2023).
Financial, technological and time constraints
To research chemotherapeutic drugs and conduct experiments a significant amount of money is needed. This can become a problem if the scientist does not have the necessary funds. In addition, the cost of chemotherapeutic drugs is very high. Cancer patients most likely will not have enough money to pay for the expensive treatment.
The cost for the 28 tablets of the vismodegib oral capsule (150mg) is approximately $13,677.00 USD, which is considerably expensive for the amount received and the cost-benefit is not mutual and fair. Trastuzumab’s cost for a full course treatment is about $70,000.00 USD. One powder injection (150 mg) is around $1,650.00 USD, yet this drug has seen a higher success rate, so there is an increased cost-benefit but is still expensive.
The time taken to produce the Vismodegib drug was a span of 8 years, with the cohort study lasting from July 2011 till September 2019. The drug is only prescribed to seven hospitals, which tells us that even after production, to refine, improve and ensure its safety will take up a lot of time (Broderick, 2016).
Due to limitations in understanding of CSC and technology, it is restraining to produce drugs to apoptose them. However, we can argue that there is nanotechnology, but also that they have not understood how CSC’s mechanism works due to the lack of knowledge and technological devices to zoom into the cells.
ABC transporters
ATP binding cassette proteins, also known as ABC transporters, such as P-glycoprotein (P-gp) and subfamily B member 1 (ABC B1) or breast cancer resistance protein (BCRP), are located in the cell membrane. They are responsible for regulating chemical compounds as well as the absorption, excretion and distribution of them and protect cells from death by high intracellular drug concentration. These proteins interfere with the drug by decreasing its intracellular concentration, bioavailability, and blood-brain barrier (Bukowski et al., 2020).
P-gp is highly expressed in endothelial cells, which are the single layer of cells that line the inside of blood vessels, and contributes to the reduced chemo-drug penetration. The only way to overcome the efflux of drugs is to increase its concentration, which most of the time can cause overall toxicity of the system. This is key to cancer cell resistance as the increased expression of P-gp cancer cells strongly corresponds with enhanced resistance to specific drugs. Bukowski et al. (2020) states that “overexpression of P-gp has been observed in about 50% of all human cancers.” In some cancers, overexpression of P-gp is present before treatment and in others it is found after chemotherapy. This is associated with poor clinical response and MDR in people with distinguished types of cancers. P-gp not only causes efflux of the drug, but also inhibits pathways of apoptosis which prolongs cell life significantly.
Finding the combination of drugs that most effectively suppresses P-gp is a key focus for targeting MDR (Bukowski et al., 2020).
Problems with Cancer Stem Cell Research
Biomarkers
Biomarkers are small molecules present on cancer stem cells and they play a crucial role in the identification and characterization of tumours. They also pose several challenges that can hinder how they are utilised. One of the few challenges that biomarkers cause is the lack of biological knowledge that scientists possess about how the biomarkers react with different drug treatments. This was highlighted in a workshop by J National Cancer Institute where they ran two case studies with different clinical study design strategies for evaluating a targeted therapy and its companion predictive biomarker action (Walcher, 2020). They concluded that more research is required to further their understanding of the biology associated with the chosen biomarkers, including their role in tumour behaviour and their interplay with the drugs mechanisms of action (Walcher, 2020). This lack of knowledge also prevents scientists from understanding how effective or ineffective the treatments that they have developed are. For example, are the drugs currently being effective, or do they need to be used in combination with other drugs, or do they have to develop a new drug/treatment that is able to target biomarkers.
Another problem faced with biomarkers is dynamic expression. Dynamic expression refers to the changing levels of biomarkers in cancer stem cells over time and in response to various environmental cues (Han, 2022). Dynamic expression can contribute to drug and treatment resistance that is developed by cancer cells; this is because this change due to dynamic expression in turn causes the cancer cell to become less responsive to the targeted therapies, which rely on specific biomarker interactions. This further highlights the needs of further biological knowledge needed to gain a deeper understanding of biomarkers and the ability to develop effective targeted therapies to target cancer.
Clinical Trials
Clinical trials are an essential part of medical research, especially in the case of cancer. Clinical trials allow scientists to evaluate new treatments, which allows them to improve the outcomes they will have when used on patients. Having said this, clinical trials still encounter different problems which can hinder progress and prevent the evaluation of treatments. Ethical concerns are a big factor, which prevent clinical trials from taking place. This is because some targeted cancer stem cell therapies may induce unknown side effects or unpredictable outcomes, thus causing individuals to opt out of participating in these trials. As a result, this does not allow scientists to gauge the effectiveness of the treatment. According to an article by the national library of medicine, the risks of innovative stem cell-based interventions include ‘tumour formation, immunological reactions, unexpected behaviour of the cells, and unknown long-term health effects’ (Lo and Parham, 2009). Scientists need to be able to create a balance between scientific advancements and patient well-being, meaning that even more rigorous pre-clinical testing would be required to be done before proceeding onto human trials. According to Cancer Research UK (2010), there are likelihoods of either unexpected or serious side effects. But there is also the risk that an experimental treatment could cause harm.This means that regardless of proper testing or not, some treatments can and will lead to adverse side effects in patients, which will discourage patients from participating. Thus, this slows down the rate at which new treatments are developed and then implemented into the healthcare systems.
Tumour evolution
Tumour evolution is one of the most formidable challenges that cancer research faces, which refers to the active process when cancer stem cells develop genetic and phenotypic changes over time, leading to further development of the tumour inside a patient (Davis, 2017). These changes can arise due to errors during DNA replication, and the accumulation of these changes can lead to the formation of subpopulations of cells with very different characteristics to the rest of the cells in the tumour – causing resistance against specific treatments. Casás-Selves and DeGregori (2011) state that, ‘cancer development within an individual is also an evolutionary process, which in many respects mirrors species evolution. Species evolve by mutation and selection acting on individuals in a population; tumours evolve by mutation and selection acting on cells in a tissue’ . Therefore, tumours are always prone to change like any other organism, so creating an effective treatment against various tumours is a challenge since different subpopulations of cells within the same tumour could react differently to the same treatment. Tumour evolution is not easy to study and observe either as patients cannot ethically be biopsied at multiple time points during the progression of the disease (Davis and Gao et al., 2017). Without being able to track the different changes that occur inside the tumour, scientists will not be able to gain sufficient knowledge to be able to create an effective treatment against it. This further highlights the need for technological advancements in cancer research. Moreover, each tumour develops differently inside each different person, thus meaning that even if an effective treatment is developed against one type of tumour in one person, it may not be effective against the same type of tumour in a different person. This would mean there would be additional costs in developing and researching these new types of tumours, not to mention the time and money required for clinical testing of the treatment. Overall, it is a substantial challenge that has to be overcome.
CONCLUSION
In summary, cancer’s multifaceted nature is a result of the disruption in fundamental cellular processes, underpinned by genetic mutations, environmental exposures and choices in an individuals’ lifestyle. Understanding the differences between cancer stem cells and normal stem cells holds promise for the development of cancer treatments, specifically targeting the cancer stem cells. Whilst both cancer stem cells and normal stem cells exhibit properties, such as self renewal, differentiation and growth, CSC has the ability to drive cancer, whilst normal stem cells are in part a contribution for healthy maintenance of tissues (Rahman et al., 2016).
As refined as modern cancer treatment strategies are in restraining the malignant spread of tumours, like chemotherapy, radiotherapy and CAR T-cell therapy, it is proven that to ultimately exterminate cancer cells, apoptosis to CSCs has to be induced. Breakthroughs on anti-CSC drugs have been made, like trastuzumab, vismodegib + sonidegib and metformin, securing a place in the market after reassuring its beneficence. Yet, refinements are to be made, including improving the success rate of cancer clearance by introducing stronger, targeted drug prescriptions, and reducing the severity of side effects caused. Guidance to increase efficiency of treatments can be sought from targeting the key mechanisms that regulate stemness and growth of the CSC, like the signalling pathways (Hedgehog and Wnt), the protein complex YAP1 and ABC transporters. To be able to constitute a blockage or discontinuance in these complexes can help to cut off signals for proliferation, metastasis and anti-apoptosis, which are the major factors of CSC survival.
Multidrug resistance (MDR) is one of the leading causes of chemotherapy and radiotherapy failure. The mechanisms of MDR are elevated metabolism of xenobiotics, which are enzymes that metabolise and detoxify the chemotherapeutics, increased DNA repair capacity, and microenvironmental growth factors. Another important mechanism is genetic factors, such as amplifications, epigenetic alterations and gene mutation, which ends up benefitting the CSCs. ABC transporters efflux the chemotherapeutic drug out of the cells through two proteins called P-gp and ABC B1 or BCRP. Heterogeneity of the tumour is the idea that there are many different cell types in a single tumour. The biopsy may not accurately represent the majority of the tumour, causing an ineffective drug to be used, ultimately strengthening the cancer. High level of funding and investment is needed to carry out the necessary research and experiments to allow for further technological advances in this field. This may, as a result, cause treatment to be increasingly expensive for patients, making it less readily accessible for the majority of people.
Yet, biomarkers, clinical trials and tumour evolution are substantial challenges that cancer research encounters. The constant evolution and mutation of biomarkers leads them to becoming less responsive to certain types of treatments, and thus leading to the resistance of treatments within a tumour. Clinical trials can lead to various side effects in the volunteers taking part, regardless of vigorous testing and safety measures put in beforehand. This leads to volunteers opting out and thus slowing down the overall development of the treatment.
Finally, tumour evolution is one of the more prominent issues facing cancer research as the tumour can evolve over time inside the person, leading to different subpopulations of cells being resistant to the same treatment that is being given. This evolution can vary from person to person, which means current treatments could be registered as ineffective.
To combat this, there should be an increased investment in developing scientific and medical knowledge of cancer stem cells and their biomarkers, as well as how they can be targeted more effectively. To tackle the issue with clinical trials, scientists should consider conducting testing on other mammals, such as mice, before proceeding to testing on humans. This could help them to identify potential side effects earlier. Finally, there should be more research done into the different types of ways that tumours can evolve and how different therapies can be developed to tackle them.
Bibliography
Akwii, R.G., Sajib, M.S., Zahra, F.T. and Mikelis, C.M. ,(2019). Role of Angiopoietin-2 in Vascular Physiology and Pathophysiology. Cells, 8(5), p.471. Doi: https://doi.org/10.3390/cells8050471
Allott, A., et al.,(2014). Biology : Oxford IB diploma programme. Course Companion (2014th ed.). Oxford University Press.
Begicevic, R.-R and Falasca, M.(2017). ABC Transporters in Cancer Stem Cells: Beyond Chemoresistance. International Journal of Molecular Sciences, 18(11), p.2362. Doi: https://doi.org/10.3390/ijms18112362.
Broderick, J. (2016). Large Trial Confirms Vismodegib Benefit in Basal Cell Carcinoma. [online] OncLive. Available at: https://www.onclive.com/view/large-trial-confirms-vismodegib-benefit-in-basal-cell-carcinoma.
Bukowski, K. et al., (2020). Mechanisms of Multidrug Resistance in Cancer Chemotherapy. International Journal of Molecular Sciences, [online] 21(9), p.3233. Doi: https://doi.org/10.3390/ijms21093233.
Cameron, D. (2015). How to Kill a Protein. [online] hms.harvard.edu. Available at: https://hms.harvard.edu/news/how-kill-protein
Cancer Council Victoria. (1900). Ways alcohol causes cancer – Cancer Council Victoria. Cancervic.org.au. https://www.cancervic.org.au/cancer-information/preventing-cancer/limit-alcohol/how-alcohol-causes-cancer#:~:text=of%20other%20carcinogens.
Cancer Research UK (2021). Trastuzumab (Herceptin) | Cancer information | Cancer Research UK. [online] www.cancerresearchuk.org. Available at: https://www.cancerresearchuk.org/about-cancer/treatment/drugs/trastuzumab.
Cancer. (2010). Nih.gov; Centers for Disease Control and Prevention (US). https://www.ncbi.nlm.nih.gov/books/NBK53010/
Casás-Selves, M. et al., (2011). How Cancer Shapes Evolution and How Evolution Shapes Cancer. Evolution: Education and Outreach, [online] 4(4), pp.624–634. Doi: https://doi.org/10.1007/s12052-011-0373-y.
Chen, W., Dong, J., Haiech, J., Kilhoffer, M.-C. and Zeniou, M. (2016). Cancer Stem Cell Quiescence and Plasticity as Major Challenges in Cancer Therapy. Stem Cells International, 2016, pp.1–16. doi:https://doi.org/10.1155/2016/1740936.
Choi, Y. (2013). Consumption of red and processed meat and esophageal cancer risk: Meta-analysis. World Journal of Gastroenterology, 19(7), 1020. https://doi.org/10.3748/wjg.v19.i7.1020
Cochrane, C. et al., (2015). Hedgehog Signaling in the Maintenance of Cancer Stem Cells. Cancers, 7(3), pp.1554–1585. Doi: https://doi.org/10.3390/cancers7030851.
Coffey, R.J.et al., (1992). Roles for transforming growth factor-alpha in gastric physiology and pathophysiology. The Yale Journal of Biology and Medicine, [online] 65(6), pp.693–623. Available at: https://www.ncbi.nlm.nih.gov/pmc/articles/PMC2589761/#:~:text=Transforming%20growth%20factor%20alpha%20(TGF
D’Orazio, J.et al., (2013). UV Radiation and the Skin. International Journal of Molecular Sciences, 14(6), 12222–12248. https://doi.org/10.3390/ijms140612222
Daugan, M. et al., (2016). Metformin: An anti-diabetic drug to fight cancer. Pharmacological Research, 113, pp.675–685. Doi: https://doi.org/10.1016/j.phrs.2016.10.006.
Dave, B., Mittal, V., Tan, N.M. and Chang, J.C. (2012). Epithelial-mesenchymal transition, cancer stem cells and treatment resistance. Breast Cancer Research, [online] 14(1). doi:https://doi.org/10.1186/bcr2938.
Davis, A. (2017). Tumor evolution: Linear, branching, neutral or punctuated? Biochimica et Biophysica Acta (BBA) – Reviews on Cancer, 1867(2), pp.151–161. doi:https://doi.org/10.1016/j.bbcan.2017.01.003.
Davis, A.et al., (2017). Tumor evolution: Linear, branching, neutral or punctuated? Biochimica et Biophysica Acta (BBA) – Reviews on Cancer, 1867(2), pp.151–161. doi:https://doi.org/10.1016/j.bbcan.2017.01.003.
Davis, J. (2020). What is the Hedgehog Signaling Pathway? [online] News-Medical.net. Available at: https://www.news-medical.net/life-sciences/What-is-the-Hedgehog-Signaling-Pathway.aspx.
De Smaele et al., (2010). Vismodegib, a small-molecule inhibitor of the hedgehog pathway for the treatment of advanced cancers. Current Opinion in Investigational Drugs (London, England: 2000), [online] 11(6), pp.707–718. Available at:https://pubmed.ncbi.nlm.nih.gov/20496266/#:~:text=Vismodegib%20suppresses%20Hh%20signaling%20by%20binding%20to%20and
Drugs.com (2018). Metformin Side Effects. [online] Drugs.com. Available at: https://www.drugs.com/sfx/metformin-side-effects.html.
Duan, C.et al., (2023). Overcoming Cancer Multi-drug Resistance (MDR): Reasons, mechanisms, nanotherapeutic solutions, and challenges. PubMed, 162, pp.114643–114643. doi:https://doi.org/10.1016/j.biopha.2023.114643.
Duffy, A.M. et al., (2013). Vascular Endothelial Growth Factor (VEGF) and Its Role in Non-Endothelial Cells: Autocrine Signalling by VEGF. [online] www.ncbi.nlm.nih.gov. Landes Bioscience. Available at: https://www.ncbi.nlm.nih.gov/books/NBK6482/#:~:text=Vascular%20endothelial%20growth%20factor%20(VEGF)%20is%20a%20potent%20angiogenic%20factor.
El-Sayes, N.et al., (2021). Tumor Heterogeneity: A Great Barrier in the Age of Cancer Immunotherapy. Cancers, [online] 13(4), p.806. doi:https://doi.org/10.3390/cancers13040806.\
Han, C. (2022). Development of a dynamic network biomarkers method and its application for detecting the tipping point of prior disease development. PubMed, 20, pp.1189–1197. doi:https://doi.org/10.1016/j.csbj.2022.02.019.
John, E. M et al., (2011). Meat Consumption, Cooking Practices, Meat Mutagens, and Risk of Prostate Cancer. Nutrition and Cancer, 63(4), 525–537. https://doi.org/10.1080/01635581.2011.539311
Kahn, M et al., (2014). The role of the Wnt signaling pathway in cancer stem cells: prospects for drug development. Research and Reports in Biochemistry, p.1. doi:https://doi.org/10.2147/rrbc.s53823.
Kong, D., Li, Y., Wang, Z. and Sarkar, F. (2011). Cancer Stem Cells and Epithelial-to-Mesenchymal Transition (EMT)-Phenotypic Cells: Are They Cousins or Twins? Cancers, 3(1), pp.716–729. doi:https://doi.org/10.3390/cancers30100716.
Li, S. et al., (2015). Cancer stem cells, lymphangiogenesis, and lymphatic metastasis. Cancer Letters, 357(2), pp.438–447. doi:https://doi.org/10.1016/j.canlet.2014.12.013.
Li, Y. (2021). Drug resistance and Cancer stem cells. Cell Communication and Signaling, 19(1). doi:https://doi.org/10.1186/s12964-020-00627-5.
Lo, B. et al., (2009). Ethical Issues in Stem Cell Research. Endocrine Reviews, [online] 30(3), pp.204–213. doi:https://doi.org/10.1210/er.2008-0031.
Matsumoto, K. (2004). Hepatocyte Growth Factor – an overview | ScienceDirect Topics. [online] www.sciencedirect.com. Available at: https://www.sciencedirect.com/topics/neuroscience/hepatocyte-growthfactor#:~:text=Hepatocyte%20growth%20factor%20(HGF)%20is%20among%20a%20group%20of%20factors
National Cancer Institute (2020). Types of Cancer Treatment. [online] National Cancer Institute. Available at: https://www.cancer.gov/about-cancer/treatment/types.
National Cancer Institute. (2017). Asbestos Exposure and Cancer Risk Fact Sheet. National Cancer Institute; Cancer.gov. https://www.cancer.gov/about-cancer/causes-prevention/risk/substances/asbestos/asbestos-fact-sheet
Ng, T.L et al., (2005). Nuclear beta-catenin in mesenchymal tumors. Modern Pathology: An Official Journal of the United States and Canadian Academy of Pathology, Inc, [online] 18(1), pp.68–74. doi:https://doi.org/10.1038/modpathol.3800272.
Pierce, G.F. et al., (1991). Role of platelet-derived growth factor in wound healing. Journal of Cellular Biochemistry, 45(4), pp.319–326. doi:https://doi.org/10.1002/jcb.240450403.
Proietto, M. et al., (2023). Tumor heterogeneity: preclinical models, emerging technologies, and future applications. Frontiers in Oncology, 13. doi:https://doi.org/10.3389/fonc.2023.1164535.
Qu, J. et al., (2021). Low-density lipoprotein receptor-related protein 1 (LRP1) is a novel receptor for apolipoprotein A4 (APOA4) in adipose tissue. Scientific Reports, [online] 11(1), p.13289. doi:https://doi.org/10.1038/s41598-021-92711-0.
Rahman, M et al., (2016). Stem Cell and Cancer Stem Cell: A Tale of Two Cells. Progress in Stem Cell, 3(02), 97. https://doi.org/10.15419/psc.v3i02.124
Sever, R et al., (2015). Signal Transduction in Cancer. Cold Spring Harbor Perspectives in Medicine, 5(4), a006098–a006098. https://doi.org/10.1101/cshperspect.a006098
Shibata, M et al., (2019). Targeting Cancer Stem Cells: A Strategy for Effective Eradication of Cancer. Cancers, 11(5), p.732. doi:https://doi.org/10.3390/cancers11050732.
Sun, X. (2023). Recent advances in access to overcome cancer drug resistance by nanocarrier drug delivery system. Cancer Drug Resistance, [online] 6(2), pp.390–415. doi:https://doi.org/10.20517/cdr.2023.16.
Walcher, L. (2020). Cancer Stem Cells—Origins and Biomarkers: Perspectives for Targeted Personalized Therapies. Frontiers in Immunology, [online] 11(1280). doi:https://doi.org/10.3389/fimmu.2020.01280.
Yu, Y. et al., (2023). Metformin and Cancer: Solutions to a Real-World Evidence Failure. Diabetes Care, [online] 46(5), pp.904–912. doi:https://doi.org/10.2337/dci22-0047.
Yu, Z., et al., (2012). Cancer stem cells. The International Journal of Biochemistry & Cell Biology, 44(12), 2144–2151. https://doi.org/10.1016/j.biocel.2012.08.022
Zanoni, M., Bravaccini, S., Fabbri, F. and Arienti, C. (2022). Emerging Roles of Aldehyde Dehydrogenase Isoforms in Anti-cancer Therapy Resistance. Frontiers in Medicine, [online] 9, p.795762. doi:https://doi.org/10.3389/fmed.2022.795762.
Zhou, Y et al., (2022). Wnt signaling pathway in cancer immunotherapy. Cancer Letters, [online] 525, pp.84–96. doi:https://doi.org/10.1016/j.canlet.2021.10.034.